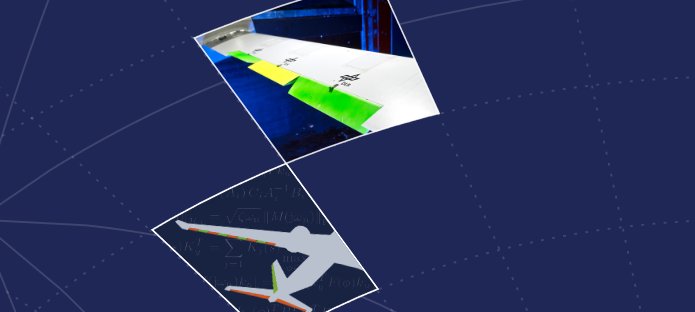
Politecnico di Milano - Aula T.0.1 (Edificio 13)
Via Bonardi 9, 20133 Milano
Speaker: Prof. Dr. Manuel Pusch (University of Applied Sciences Munich)
Contatti: Prof. Lorenzo Fagiano | lorenzo.fagiano@polimi.it
Highly fuel-efficient aircraft designs often suffer from undesired aeroelastic effects, or more specifically, from interactions of structural dynamics and aerodynamics that can cause large structural loads or even instabilities. To counteract these adverse effects, active control of critical aeroelastic modes during flight is becoming increasingly important. To this end, more and more sensors and control surfaces are installed on aircraft, enabling an improved closed-loop performance at the cost of a more complicated controller design.
In this talk, two novel modal control approaches for linear time-invariant systems are presented which are based on blending, i.e., weighting and summing up, of control inputs and measurement outputs. In doing so, the original multivariable control problem for controlling a specific targeted mode is reduced to a “single-input single-output” one. In the first approach, input and output blending vectors are computed such that controllability and observability of the targeted mode are maximized in terms of the H2 norm. The second control approach searches for blending vectors which allow the poles of the targeted mode to be shifted to desired locations in the complex plane with a minimum static feedback gain. In both approaches, the blending vector design problem is reformulated to make the controller synthesis independent of the actual number of inputs and outputs. Taking into account explicit mode decoupling constraints, it is ensured that the residual system dynamics are not affected and that each targeted mode can be controlled independently. To further consider actuator constraints like saturation or faults, an enhancement of the proposed control approaches is presented making use of real-time control allocation.
The effectiveness and applicability of the proposed control methods are validated based on two realistic aeroelastic systems. In the first application, a flutter suppression controller is designed to increase the operational velocity range of an unmanned aerial vehicle by stabilizing undesired interactions of structural dynamics and aerodynamics. The second application considers an experimental flexible wing for which a gust load alleviation controller is designed taking into account actuator constraints. The great potential of the proposed control approaches is not only demonstrated by comprehensive linear and nonlinear simulations but also confirmed in wind tunnel and flight test campaigns.